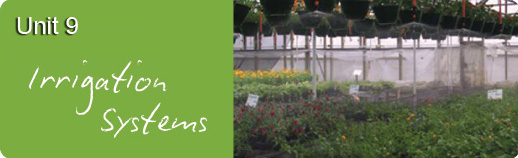
Section 3: Correcting Water Quality Problems
pH and Alkalinity
Acid injection may be used to adjust the pH of the water as well as the alkalinity level. Enough acid may be added to either lower the alkalinity down to the desired level (with little impact on pH since the carbonates and bicarbonates neutralize the acid and buffer the water pH) or enough may be added to neutralize the alkalinity and lower the pH to a desired level.
Acidification reduces the alkalinity level of water by neutralizing the bicarbonates and carbonates (as well as other bases) in the water. Acids most commonly used to neutralize alkalinity in water to be used for greenhouse crops production include citric acid (either 99.5% granular or 50% liquid), nitric acid (61.4% or 67% liquid), phosphoric acid (75% or 85% liquid), and sulfuric acid (35% or 93% liquid).
Each of these acids has advantages and disadvantages and the most appropriate one to use depends upon the actual water alkalinity level, the volume of water that needs to be adjusted and the mineral nutrition program being used.
Citric acid is effective at reducing water alkalinity but it is expensive and also has little residual effect on pH. However, citric acid does not provide mineral nutrients and does not interact with other fertilizer salts. Therefore, it is most useful where the alkalinity or pH of a fertilizer stock solution needs to be adjusted or where water pH needs to be adjusted for mixing of pesticides or where only small corrections are needed. Although it can cause minor skin and eye irritation, citric acid is relatively safe and easy to use.
Nitric acid is very effective at reducing water alkalinity but it can be dangerous and difficult to use (proper storage and safe handling practices must be followed). Nitric acid should not come in contact with the skin or eyes and care should be taken not to breathe the fumes. Appropriate protective gear should be worn when dealing with nitric acid. Nitric acid also provides nitrogen to the irrigation water (and thus to the mineral nutrition program). As is shown in Table 2, a fluid ounce (1.0 fl. oz.) of 67% nitric acid in 1000 gallons of water will provide 1.64 ppm nitrogen.
Sulfuric acid is very commonly used to reduce water alkalinity or to adjust water pH. It is relatively low cost. Although it can cause skin and eye irritation, it is less dangerous and less caustic than nitric acid. Sulfuric acid also provides sulfur to the irrigation water. As shown in Table 2, a fluid ounce (1.0 fl. oz.) of 35% sulfuric acid in 1000 gallons of water will provide 1.14 ppm sulfur. Excessive levels of sulfur from the use of too much sulfuric acid can result in the tie-up (make unavailable for uptake by the plant) of calcium in the substrate (insoluble calcium sulfate, or gypsum, forms). Sulfuric acid should always be stored and handled properly and appropriate protective gear should be worn when dealing with sulfuric acid.
Phosphoric acid is also commonly used to adjust water alkalinity and pH. However, it is a weaker acid than nitric and sulfuric acid. It is caustic and can cause skin and eye irritation, so proper storage and handling should be followed. Phosphoric acid provides phosphorus to the irrigation water. As is shown in Table 2, a fluid ounce (1.0 fl. oz.) of 75% phosphoric acid in 1000 gallons of water will provide 2.88 ppm phosphorus. In cases where the water alkalinity is very high, a large amount of phosphoric acid would be required to adjust the water alkalinity to the desired level. This would result in the supply of a large amount of phosphorus to the irrigation water. In such a case, the level of phosphorus in the irrigation water might exceed the recommended level. High levels of phosphorus can cause stretching or elongation of young plants and very high phosphorus levels can cause the tie-up of iron. Therefore, where large water alkalinity level corrections are required, it may be preferable to use sulfuric or nitric acid to adjust the water alkalinity level.
The amount of acid to be injected depends upon the acid, the water alkalinity and the target alkalinity level (or the targeted pH). Table 2 lists the amount of various acids to add to 1000 gallons of water for each meq.L-1 of alkalinity in the water to adjust the final water pH to 5.8 (alkalinity is neutralized and the pH lowered). These values can be used a general recommendation but since the starting pH of the water is not included in the calculations of these values, the exact amount of acid required may vary slightly and greenhouse managers should conduct trials to determine the exact response of their water to acid injection.
In many cases, a greenhouse manager will only want to reduce the water alkalinity to an acceptable level but not neutralize all of the carbonates and bicarbonates (and other bases) in the water as shown in the above example. In this case, the desired water alkalinity (expressed as meq.L-1) is subtracted from the actual water alkalinity. This gives the amount of alkalinity that must be neutralized. For every 1.0 meq.L-1 that needs to be neutralized, approximately 7.0 fl. oz of 85% phosphoric acid or 3.5 fl. oz of 93% sulfuric acid are required per 1000 gallons of water. For example, if the water has an alkalinity level of 2.8 meq.L-1 and the desired level is 1.0, then 1.8 meq.L-1 must be neutralized. To achieve this, 12.6 fl. oz. of 85% phosphoric acid or 6.3 fl. oz of 93% sulfuric acid would need to be added per 1000 gallons of water.
The above method does not account for the pH of the water and differences in the actual alkalinity values in water and thus provides only a general recommendation. Another method (and more exact) to determine how much acid is required to reach a target alkalinity level or pH is to use the North Carolina State University Alkalinity calculator. This software allows the greenhouse manager to enter the pH and alkalinity of the water source and the desired water alkalinity or water pH. The software calculates the amount of each type of acid required, the amount of mineral nutrients provided and a cost estimate.
Many commercial soil testing laboratories will also test water quality and will provide greenhouse managers with recommendations regarding acid injection for any corrections to alkalinity or pH required.
Caution should always be taken when using acids. Small amounts of acid should be added to larger volumes of water (NOT water to acid). As discussed below under injectors, injectors used for acid injection must be acid compatible. Acid should be injected into the water prior to the injection of fertilizers.
In addition to acid injection, water alkalinity may be reduced by using blended water. In this case low alkalinity water (such as rainwater) is blended with or added to a water source that has an undesirably high alkalinity level. The two water sources are blended together at a ratio that results in water with a desirable alkalinity level.
In cases where water alkalinity is only slightly higher than the desired level, acidic fertilizers might be used to offset or counter the increase in pH caused by the alkalinity of the water. This subject is discussed in more detail in the “Mineral Nutrition” learning unit.
Table 2. Amount of various acids required to neutralize alkalinity and lower water pH to 5.8. |
|||
Acid |
Concentration |
Amount of acid to add to 1000 gallons of water for each meq.L-1 of alkalinity to create a water pH of 5.8z |
Concentration of mineral nutrient provided for each fluid once added to 1000 gallons of water |
Citric acid |
99.5% (w/w) granular |
9.1 |
- |
Citric acid |
50% (w/w) liquid |
14.5 |
- |
Nitric acid |
67% (w/w) liquid |
6.6 |
1.64 |
Phosphoric acid |
75% (w/w) liquid |
8.1 |
2.88 |
Sulfuric acid |
35% (w/w) liquid |
11.0 |
1.14 |
z Does not take into account the pH of the water so actual amount required may vary. |
Salinity (E.C.)
High water E.C. levels are a common problem in many areas of the U.S. and world. The first possible method of correcting a high E.C. level in irrigation water is to develop an alternative source of irrigation water that has an acceptable E.C. If a reliable source of acceptable E.C. water is available, it should be used for irrigation. If only small amounts (insufficient to meet the entire need of the greenhouse operation) of low E.C. water are available, the high and low E.C. water sources can be blended to achieve an acceptable E.C. level. Some greenhouse managers collect rainwater to blend with other sources of water that may have a high E.C. In a some cases, greenhouse managers have used reverse osmosis (R.O.) units to produce "pure water" that can be blended with other water sources. These units are expensive to operate, but they may be an option depending upon the operation and the volume of water required. Never use a water softener for greenhouse irrigation water. These systems replace calcium and magnesium compounds with sodium and a high sodium level in the water can cause serious problems during crop production.
Phytotoxic ions
The major method of correcting the problem of having one or more specific ions in the water at a higher than acceptable level is to select a water source low in potentially phytotoxic ions or to blend water as described under the salinity section.
If high iron is a problem, the water may need to be aerated to cause iron to precipitate out of the water, the water may need to be filtered, or sequestering agents (i.e. Aqua-Solv) may be added to the water to precipitate out the iron.
Bacteria, fungi and algae
Greenhouse mangers may need to disinfest (kill microorganisms such as bacteria and fungi) water used for greenhouse irrigation. This may be because fresh water sources such as ground water or water from ponds or lagoons may contain algae and plants pathogens (i.e. fungi, bacteria or viruses) or because recollected and recirculated water or fertilizer solutions (i.e used in ebb-and-flow benches, Dutch trays or flood floors) are being used which might spread potential disease causing organisms around the greenhouse operation. There are numerous methods for disinfesting greenhouse irrigation water and recirculated fertilizer solutions and these are discussed below.
Ultraviolet
Ultraviolet light (UV) is a common method used by greenhouse managers to disinfest irrigation water and recirculated fertilizer solutions. Ultraviolet light treatment has proven to be effective at controlling many plant pathogens including Pythium, Fusarium, Phytothera, and Alternaria. In this system, water is passed over UV light-generating tubes which generate UV-C (typically around 254 nm wavelength) light. The UV light kills algae and plant pathogens. A limitation with using UV light is that although it can penetrate approximately 25 cm of clean water, it may penetrate low-quality water only a few millimeters since particles in the water block the UV light. Additionally, debris or organic material in the water will block the UV light. If the UV light is blocked, some pathogens will not be killed since they are protected in the shadow of the material blocking the UV light. Therefore, water quality is an important issue when using UV light to disinfest water or fertilizer solutions. When using UV light, water or fertilizer solution is usually passed through filters to remove any debris before the solution is passed through the UV-light unit. Ultraviolet light can affect chelating agents used in fertilizer solutions. If chelating agents are to be used in a fertilizer solution that will be treated with UV light, consult with the manufacturer regarding compatibility.
The effectiveness of UV-C light for disinfestation is dependent on duration and intensity of the UV light. UV-C radiation intensity for greenhouse irrigation water is typically 100 mJ/cm2 for selective disinfection (killing most fungi and bacteria) or 250 mJ/cm2 for total disinfestation including viruses. The use of UV-C radiation is an effective treatment for disinfesting greenhouse irrigation water, but since there is no residual activity, steps must be taken to keep the water clean after treatment.
Slow Sand Filtration (Biofiltration)
Slow sand filtration relies on both physical and biological activity in controlling plant pathogens. A slow sand filter has a high surface area, which can be colonized by suppressive microorganisms. This sand medium (typically in layers of different grain sizes) also presents a physical barrier to the passage of spores of fungal plant pathogens and nematodes but not to bacteria or viruses. The effectiveness of slow sand filtration is debated, and it is most commonly recommended as a pre-treatment for the other sanitation technologies such as UV light, ozonation and chlorination since particulates reduce the efficiency of these treatments.
Heat Treatment
Heat treatment was more commonly used in Europe to disinfest greenhouse water than in the U.S. Water temperature should reach 95°C (203°F) for at least 30 seconds for effective control of pathogens. A major disadvantage associated with this method is the high cost of heating the water.
Ultra-Filtration
Ultra-filtration relies on fine membrane filters that contain small pores which physically filter the suspended solids as well as fungi, bacteria and viruses. This kind of filtration completely eliminates the water pathogens, but can be very expensive. With this method, pre-filtration (i.e. through sand columns) is required and problems may arise from the filter pores becoming blocked over time. Filters with different pore sizes have been tried (0.05 μm -0.25μm). However, cost and clogging issues have limited the use of this technology in most greenhouse applications.
Ozone
Ozone treatment is more expensive in capital and operating costs than other technologies. Ozone (O3) occurs naturally during thunderstorms where oxygen (O2) comes into contact with the strong electrical field caused by lightening. Ozone used to disinfest greenhouse irrigation water is produced by ozone generators that also use a strong electrical field to generate ozone. The ozone is then added to the water by bubbling it in the water or more often by injecting it under pressure into the water in a pressurized tank (this results in more ozone being absorbed by the water). The ozone rapidly breaks down into dissolved oxygen and hydroxyl ions (OH-) as it reacts with impurities in the water. The capacity of ozone to disinfest water is affected by organic matter present, water pH, water E.C., and amount and type of iron chelates in the water. The rate of ozone breakdown increases at high water pH and its effectiveness is reduced by organic matter and mineral salts in the water. Water temperature also affects the half-life of ozone with the half-life decreasing as water temperature increases. Ozone has been shown to reduce the concentration of available iron in the water or fertilizer solution.
Recommended ozone concentrations vary depending upon the source of the information. One recommendation was for ozone to be injected into greenhouse irrigation water at 0.5 to 1.5 ppm. However, another recommendation was for residual levels of ozone not to exceed 1.0 ppm to avoid phytotoxicity. The difference may be that the first recommendation is the initial injection rate where the later is the ozone concentration after the injected ozone destroys pathogens, algae and other organic matter in the water. It also has been noted in published reports that 0.2 ppm ozone exposure for 30 minutes will destroy biofilms (organic layer build up of bacteria and algae) and that a residual concentration of 0.01 to 0.05 ppm ozone will control algae.
For optimum results, the pH of the water should be approximately 4.0 with a contact time of one hour. Because of the required exposure time, ozone is typically injected into a water storage system and should be maintained under pressure to prevent off-gassing of the ozone. In clean water, dissolved ozone can be measured with by the water's oxidation-reduction potential (ORP), but, with the strong oxidizing power of ozone, water with moderate turbidity can result in ORP values far below expected levels. One of the primary advantages of ozone disinfestation is that no corrosive chemicals are involved. Yet, there is little residual disinfesting potential after treatment. Also, ozone may react with some fertilizers oxidizing iron, manganese and sulfides.
Chlorination
Chlorination is effective at killing a range of plant pathogens. It works rapidly, and is relatively inexpensive to utilize. However, it is active over a smaller pH range than some other methods. Chlorination is usually carried out by injecting metered amounts of sodium hypochlorite solution, calcium hypochlorite solution or chlorine gas. The difficulty with effective chlorination is that the amount of chlorine required depends on the impurities, primarily organic matter, in the water. To determine whether chlorination is effective, the residual free chlorine needs to be monitored regularly.
Chlorine activity may be measured and reported as free residual chlorine or total chlorine in ppm. A typical recommended rate for disinfesting greenhouse irrigation water has been to inject enough chlorine to have 5.0 ppm at the well head with a residual level (maintained in the water after initial oxidation of organic matter in the water) of 0.5 to 2.0 ppm (for continuous application). However, chlorine exists in water as HOCl (hypochlorous acid) or OCl-1 (hypochlorite). Hypochlorous acid is a strong, fast acting oxidizer, whereas, hypochlorite is much weaker. As the pH of the solution increases, HOCl converts to its ionic form OCl-1. Therefore, measuring the total chlorine of a solution does not necessarily indicate the oxidizing strength of a solution (the actual disinfesting strength depends on the concentration of chlorine and the form). In order to maintain free chlorine in its most active form, the solution pH should be maintained between 7.4 and 7.6 A better measurement of the disinfesting potential of chlorine in water (as well as other oxidizing agents such as fluorine, bromine and ozone), is the water’s oxidation-reduction potential (ORP with units of milliVolts). Data from many sources has established that 650-700 milliVolts ORP as the minimum threshold for typical anti-bacterial activity. Numerous meters are available that measure both free chlorine in water as well as the ORP of water. Some of these meters are designed to be placed in the irrigation line while others are simple hand-held devices.
Chlorine for disinfesting irrigation water is available in four common forms including chlorine gas, sodium hypochlorite, calcium hypochlorite and chlorine dioxide (discussed below). Each has advantages and disadvantages. Chlorine gas is probably the cheapest form of chlorine available but it requires on-site injection equipment. It is most active between the pH 6.0 to 7.5. Chlorine gas requires specific pH and concentration control, and it is highly corrosive if improperly handled. Chlorine gas is also dangerous and requires alarms and scrubbing technology in the event of a spill. Sodium hypochlorite is the active ingredient in standard household bleach. Household bleach typically contains 3% to 6% NaOCl, while industrial bleaches are typically 10% to 12% NaOCl. Sodium hypochlorite dissociates in water to release hypochlorous acid and there is a concomitant formation of sodium hydroxide. Sodium hydroxide is corrosive to metals so specialized equipment and pumps are required with its use. Because of its corrosiveness, sodium hypochlorite is not often used for greenhouse irrigation water disinfestation. Calcium hypochlorite is a dry form of chlorine and when dissolved in water, is an effective disinfestant that eliminates bacteria, algae, slime, fungi and other microorganisms. Calcium hypochlorite has many advantages over chlorine gas and sodium hypochlorite. Calcium hypochlorite is much safer to handle compared to both chlorine gas and sodium hypochlorite. It is easier to store, and it is not as corrosive and is less harsh on equipment. Calcium hypochlorite is typically sold in tablets and some form of tank must be used for the tablets to dissolve into water. The tanks are designed so that the tablets break down at a consistent rate, releasing a controlled amount of chlorine into the water flow (i.e. Accu-Tab System).
There is a danger of phytotoxicity to plants if the residual free chlorine levels rise too high. Health hazards are a concern and safety precautions must be taken when handling the chlorination chemicals and also when storing the products.
Chlorine dioxide
Chlorine dioxide can be used to kill algae, bacteria, viruses and fungi in greenhouse irrigation systems. Chlorine dioxide is a yellowish gas which is produced on site by combining hydrochloric acid and sodium chlorite. Chlorine dioxide is 25 times more effective than chlorine gas as a disinfectant and is a synthetic yellowish-green gas with chlorine like odor. Chlorine dioxide is unstable as a gas, but is stable and soluble in water. The instability of chlorine dioxide requires that it must be produced and used at the same location requiring specialized equipment. Yet, chlorine dioxide is an extremely effective biocide, disinfectant agent and oxidizer even in the presence of high organic load conditions common to recirculated greenhouse irrigation water. Compared to calcium hypochlorite, chlorine dioxide is effective over a broader pH range with maximum efficacy at pH of 8.5. Disadvantages include the cost and complexity of the system, residual build up in substrate over time and the need for appropriate storage facilities for the chemicals involved.
Bromine
Bromine is similar in action to chlorine but it is more active across a wider pH range. Bromine is created similarly to chlorine, by adding sodium bromide, a natural compound found in sea water, to sodium hypochlorite. A lower concentration of bromine is needed to effectively kill pathogens as compared to chlorine.
Iodine
Iodine is a strong fungicide and bactericide. Iodine is effective even with high organic loads in the water (dirty water) and is not affected by pH. It remains active in water with a pH between 3.0 and 8.5. Iodine has no impact on nutrients in the fertilizer solution and therefore, fertigation and sanitation can be used in combination. Monitoring of iodine levels is very important to reduce the potential for phytotoxicity to plants.
Copper ionization
Copper coils may be placed in the water or fertilizer storage tanks. An electric current is passed through the coil which results in copper ions being given off from the coil into the water. The copper acts as an effective biocide and kills most bacteria and fungi. Copper concentrations of 1.0 to 1.6 ppm are typically used and a concentration of 1.15 ppm was found effective against Pythium.