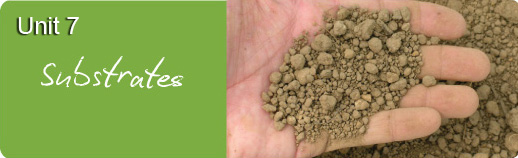
Section 2: Important Physical Properties of Substrates
Bulk density
Bulk density is the dry weight of a specific volume of a moist substrate. It is determined by measuring a specific volume of a moist substrate (usually at 50% or 60% moisture by weight) and drying the substrate in an oven until no additional moisture is lost over a 24-hour period. Bulk density is therefore usually expressed as grams per cubic centimeter (g.cm-3). However, in the U.S. it is common in the greenhouse industry for bulk density to be expressed as a moist weight per volume. In these instances, bulk density is often expressed as lbs per cubic yard or lbs per cubic foot. In the European Union, bulk density of commercial substrates is sometimes expressed as kilograms per cubic meter. Although these are not proper expressions of bulk density in the truest sense, they do provide users with an understanding of the moist weight of the root medium, and therefore, the weight that must be supported by benches or that must be shipped. Most peat-based and peat-bark-based substrates have a bulk density of approximately 0.09 - 0.16 g.cm-3 (Table 1). Although a bulk density of 0.15 - 1.3 g.cm-3 has been recommended for greenhouse substrates, the required bulk density will depend upon the crop being grown in the substrate. A certain bulk density is desirable, especially with larger or taller plant materials since the weight of the substrate will support the plant and prevent the container from falling over. However, as bulk density increases, the cost of shipping also increases. Therefore, a substrate with a high bulk density will be desirable when growing a crop such as poinsettia trees or tall foliage plants, whereas a low bulk density root substrate would be desirable for growing bedding plants in small containers or in cell packs.
Total pore space
Most common substrates to be used for the production of containerized greenhouse crops will typically have a total pore space of 75% to nearly 90% (Table 1). These figures may vary depending upon the exact specifications and proportions of the components used to formulate the substrate. However, these numbers illustrate the important point that the majority of the volume of a substrate is actually comprised of the pore spaces that exist between the solid substrate particles. Although it has been recommended that total pore space of substrates should be 70% - 90% by volume, the total pore space itself is less important than the size of the pores that make up the total pore space. It is the size of these pores, or the abundance of different sizes of pores, that will impact the very important physical properties known as air-filled pore space and water-holding capacity.
Air-filled pore space
During irrigation, when a crop is top watered, all of the pores of a substrate in a container are filled with water (saturated condition). However, immediately after irrigation, the larger pores are unable to hold water against gravity and they drain and become air-filled. At this point, being that it is holding the maximum amount of water that it is capable of holding against gravity in the container in which it has been placed, the substrate is said to be at container capacity. These air-filled pores are important because they allow for gas exchange between the roots and the outside atmosphere. Without this gas exchange, the roots would be deprived of oxygen required for respiration. If the air-filled pore space is too low, there is an increased probability of root rot occurring. Additionally, if oxygen in the substrate is too low, conditions may become anaerobic. Under anaerobic conditions ethanol and ethylene may build up in the substrate. Reduced forms of manganese and sulfur compounds may also result from these conditions. Although there are no absolute recommendations, an air-filled pore space of 10 to 20% has generally been recommended for most containerized greenhouse crops. As an example, a typical 80% sphagnum peat with 20% perlite or an 80% sphagnum peat with 20% composted pine bark (3/8 inch) will have an air-filled pore space of approximately 10% and 13% in a 4-inch container, respectively (Table 1). Increasing the amount of perlite or bark (or other large particles such as PBH or pumice) will generally increase the amount of air-filled pore space but only up to a maximum of the air-filled pore space of the aggregate being used (i.e. the perlite, pumice or bark).
As noted earlier, air-filled pore space and container capacity are defined as the condition of the substrate after drainage in a specific size container. This is because the container size, and specifically the height of the container, significantly affects the physical properties of air-filled pore space and water-holding capacity. Any substrate will have a different air-filled pore space depending upon the container size. Therefore, the air-filled pore space of a substrate should be discussed in relation to the container in which it is placed. The role of container size on air-filled pore space is explained further under water-holding capacity.
Water-holding capacity
Possibly the most important function of a substrate is to hold water (or the fertilizer solution when using a liquid fertilization program) in a form that is available for uptake by the plant. If the water-holding capacity is too low, frequent irrigation will be required to prevent water stress. However, if the water-holding capacity is too high (too many of the pores are filled with water at container capacity), the air-filled pore space may be too low. Additionally, if the water-holding capacity is too high, infrequent irrigations might be required, and in cases where a constant liquid fertilization program is being utilized, this results in infrequent fertilization and can lead to either nutrient deficiencies or the grower being forced to water too frequently in order to provide adequate fertilizer to the crop.
Water-holding capacity for substrates in containers is expressed as the percent of the volume of the substrate that is filled with water at container capacity and is expressed as a percentage of the total volume. Recommendations for the optimal water-holding capacity of a substrate vary depending upon the crop and growing conditions. However, a common recommendation has been that the substrate should have a water-holding capacity of 50% to 65%. However, many commonly utilized substrates may have higher water-holding capacities. A typical 80% sphagnum peat and 20% perlite substrate will hold approximately 68% water by volume while an 80% sphagnum peat and 20% composted pine bark substrate will hold approximately 70% water by volume (Table 1).
Not all of the water that is held by the substrate is available for uptake by plants. The water held by a substrate has traditionally been divided into easily available water, available water and unavailable water. Available water has generally been defined as water that is held at a tension of between 10 and 150 cm while easily available water has been defined as water held between 10 and 50 cm of tension. The important point from a crop production standpoint is that, although total water-holding capacity is usually what is reported for a substrate, not all water that a substrate retains is available for use by the plant.
In addition to the components and the ratio of the components, water-holding capacity, as well as the air-filled pore space of a substrate, is also a function of the container into which the substrate is placed. As the container height decreases, the water-filled pore space increases and the air-filled pore space decreases (as a percentage of the total volume of root substrate). By contrast, as the height of the container increases, the water-filled pore space decreases and the air-filled pore space increases. Thus, a given root substrate will hold more water and have less air-filled pore space when placed in a short container than when placed in a taller container. This is very similar to the phenomena seen when a sponge is saturated with water, picked up and allowed to drain. If the sponge is held so that its longest side is flat (horizontal), some of the pores of the sponge will drain and then drainage will cease. The pores that drain become air-filled. If the sponge is then turned with its longest side vertical, additional water will drain (and more of the pores will be air-filled). This is important because a root substrate that works well in a tall container, may have too high of a water-holding capacity and too low of an air-filled pore space when placed in a short container. Therefore, the container in which the substrate is being placed should be considered when designing a substrate.
Shrinkage
Shrinkage usually refers to the loss of substrate in the container over time due to decomposition of the organic components of the substrate. All organic materials used in substrates will decompose over time. However, the important issue is how fast the organic components decompose. If the organic components decompose slowly, the loss of the substrate is slow and the crop cycle may be complete before a significant reduction in the substrate volume occurs. If the substrate decomposes rapidly, the volume of the substrate will be reduced rapidly and before the crop cycle is completed, little of the organic components of the substrate might remain to fulfill the functions for which they were intended. With little substrate remaining in the container, very frequent irrigations become necessary to prevent water stress and without the weight of the substrate and the water that it holds, taller plants become prone to toppling.
Sometimes shrinkage has been used to refer to the volume loss that occurs when a substrate is allowed to dry. This is most prominent with peat-based substrates. As water is removed from the substrate through transpiration and evaporation the peat particles shrink and contract. If the substrate is allowed to become too dry, and especially if no wetting agent has been used, rewetting of the peat may be difficult.
Finally, shrinkage has occasionally been used to refer to the loss of substrate volume that occurs as a result of top watering. In this case, this loss of volume results simply from a settling of the substrate particles as water flows through the substrate. Most of this settling occurs during the first two or three irrigation cycles but it increases the bulk density and reduces the total pore space and air-filled pore space of the substrate.

